Research Highlights of the Department of Physics
HU researchers develop a light amplifier for efficient fiber optic networks
Today, optical fibers are the backbone of our information society. However, in order to transmit data over long distances using light in fiber optic networks, the light must be amplified regularly to compensate for the losses that occur. So-called repeaters are used for this purpose. One important repeater design is based on amplifying light using the laser effect. For this purpose, atoms within the optical fiber are put into an excited state. They are then stimulated by the light to emit their stored energy in the form of additional light particles. Thus, more light comes out of the laser amplifier than what goes in. Under ordinary circumstances, a laser amplifier would amplify forward and backward propagating light equally, since atoms are round particles and would therefore emit additional light particles equally in all directions. In fiber-optic networks, this can become a problem if, for example, spurious signals traveling in the backward direction are amplified. Researchers led by quantum physicist Arno Rauschenbeutel at the Humboldt-Universität zu Berlin have now demonstrated a novel way to get atoms to amplify light in an optical fiber in only one direction. To do this, they forced the light guided in the optical fiber through a taper in which the optical fiber is a hundred times thinner than a human hair. The light in such an ultrathin optical fiber then protrudes a tiny bit beyond its surface – this is known as an evanescent light field. "The interaction between the light wave and the ultrathin glass fiber changes the oscillation state of the light field. The evanescent field then rotates like the rotor of a helicopter," explains Arno Rauschenbeutel. The direction of rotation depends on whether the light in the optical fiber is moving forward or backward. In one direction, the light will oscillate clockwise; while in the other, it oscillates counterclockwise. The direction of propagation and the oscillation state of the light are thus linked. If atoms are now made to rotate using laser light and coupled to the evanescent field of the ultrathin glass fiber, it is possible to make them behave differently with respect to the two light rotation directions. "The light field in the forward direction has the same sense of rotation as the atoms and is amplified by them. The light field in the backward direction, on the other hand, which rotates the other way around than the atoms, is not affected by them," says Philipp Schneeweiß, who conducts research on the light amplifiers together with Arno Rauschenbeutel. The researchers demonstrated this effect in an experiment: They cooled a few hundred atoms to a temperature of a few millionths of a degree above absolute zero, so that they stood almost still and lined up along the ultrathin glass fiber. Although only about as many atoms were used as there are in a single insulin molecule, they still managed to amplify the light in one direction by a factor of two. In the opposite direction, however, the intensity of the light did not change. The researchers are optimistic that the demonstrated principle will soon find practical applications. In addition to fiber optic networks, it could also be employed in superconducting quantum circuits that are used in certain quantum computers. "There, it would be particularly advantageous that our approach, unlike others, does not require magnetic fields, because these do not combine well with superconductors" explains Arno Rauschenbeutel.
Abbildung 1: Artistic illustration of the nano-repeater. Light passes through an ultra-thin glass fiber and protrudes a bit beyond its surface. Atoms near the fibre are optically excited and set into clockwise rotation. Thanks to the special properties of the light field around the ultrathin glass fiber, the atoms only amplify the light when it travels from left to right. (Grafik: HU Berlin)
Publication
Atomic spin-controlled non-reciprocal Raman amplification of fibre-guided light
Sebastian Pucher, Christian Liedl, Shuwei Jin, Arno Rauschenbeutel & Philipp Schneeweiss, Nature Photonics 16, pages 380–383 (2022)
Kontakt
Arbeitsgruppe Grundlagen der Photonik
FAIR research data for the materials sciences:
The FAIRmat consortium, led by Humboldt University, describes its concept for accessible research data in the renowned journal Nature.
The lifestyle of our society is largely determined by the achievements of condensed matter physics, chemistry, and material sciences. Be it touch screens, batteries, electronics or implants - many new products in the fields of energy, environment, health, mobility, and information technology are largely based on improved or even novel materials. The enormous amounts of data produced daily in these research fields represent a new raw material - and can be considered a gold mine. This gold mine is, however, of little value if these data are not comprehensively characterized and made available. The FAIRmat consortium ("FAIR Data Infrastructure for Condensed-Matter Physics and the Chemical Physics of Solids"), led by HU professor Claudia Draxl, has set itself the goal of refining this raw material, i.e. turning data into knowledge and value. A basic building block for this is a data infrastructure that makes it possible to make data "FAIR", i.e. findable, accessible, interoperable and re-purposable. "With a "FAIR" infrastructure, data can be easily shared and explored using data analytics and artificial intelligence methods. "This approach will significantly change the way science is done today," says Claudia Draxl. In the journal Nature, the scientists describe how such a data infrastructure can be successfully implemented in the field of materials science. The article appeared on April 28, 2022 in the "Perspectives" format, in which the journal publishes forward-looking contributions that stimulate discussion and new scientific approaches. The FAIRmat consortium is part of the National Research Data Infrastructure (NFDI). The project is based on the extensive experience with the world's largest data infrastructure in computational materials science, the Novel Materials Discovery (NOMAD) Laboratory, which was co-founded by Claudia Draxl and has been online since 2014. The main challenges for FAIRmat lie in the integration of the multitude of experimental characterization techniques and material synthesis methods.
Abbildung 1: Mode of action of the FAIRmat-Consortium (Copyright: FAIRmat).
Publication
FAIR data enabling new horizons for materials research
Matthias Scheffler, Martin Aeschlimann, Martin Albrecht, Tristan Bereau, Hans-Joachim Bungartz, Claudia Felser, Mark Greiner, Axel Groß, Christoph Koch, Kurt Kremer, Wolfgang E. Nagel, Markus Scheidgen, Christof Wöll, and Claudia Draxl, Nature 604, pages 635–642 (2022)
Kontakt
Prof. Dr. Claudia Draxl Institut für Physik / IRIS Adlershof Humboldt-Universität zu Berlin Tel.: 030 2093-66363 E-Mail: claudia.draxl--at--physik.hu-berlin.de
AIP Scilight: Quantum friction could become physically measurable
Quantum friction is a contactless drag that arises when objects in vacuum at zero temperature move relative to each other. It is solely due to the modification of quantum electromagnetic fluctuations generated by the materials of the two bodies. The American Institute of Physics (AIP) has honored an article by Daniel Reiche, Francesco Intravaia, and Kurt Busch in Applied Physics Letters Photonics with an AIP Scilight, highlighting this work as particularly interesting and significant.
Experiments aiming at detecting this small drag force have so far failed to produce a clear result. In their article, the authors combine concepts of quantum field theory and nonequilibrium statistical physics, as well as atomic and condensed matter physics, to analyze the optimal conditions for the design of new experiments that should be able to make quantum friction physically and unambiguously measurable.
Figure 1: Illustration of quantum friction through quantum fluctuations near a surface.
AIP Scilight: https://doi.org/10.1063/10.0009790
Publication
Wading through the void: Exploring quantum friction and nonequilibrium fluctuations
D. Reiche, F. Intravaia, K. Busch, APL Photonics 7 (2022) 030902
Contact
Research Group Theoretische Optik/Photonik
Bremsstrahlung of black holes and neutron stars from quantum field theory
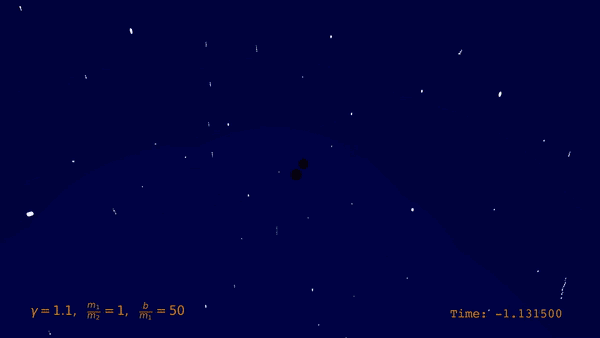
When two massive objects (black holes, neutron stars, or stars) pass each other, the gravitational interactions not only deflect their orbits, but they also produce gravitational radiation or gravitational bremsstrahlung, by analogy with electromagnetism. The resulting gravitational waves from such a scattering event were calculated to leading order in Newton's gravitational constant as early as the 1970s using traditional methods of general relativity in an extensive series of four papers. Bremsstrahlung events are still out of reach for the current generation of gravitational wave detectors because the signal is not periodic and typically less intense. Nevertheless, they are interesting targets for future searches with future Earth- and space-based observatories.
A new approach to determine these waveforms (Fig 1) and the deflections using perturbative quantum field theory methods has now been developed in the quantum field theory group led by Prof. Plefka (IRIS Adlerhof member), which proves to be much more efficient than the traditional approaches. It is based on a hybrid quantum field theory in which the black holes (or stars) are idealized as point particles interacting with the gravitational field. The calculation is then based on a systematic diagrammatic evolution using Feynmangraphs. This means that the methods originally developed for the scattering of elementary particles can now also be used in astrophysical scenarios.
This innovative method - the "Worldline Quantum Field Theory" - has recently significantly extended our understanding of this fundamental physical process in a series of three publications in Physical Review Letters. In [1], the results from the 1970s were reproduced in a much more efficient way, requiring only the calculation of three Feynmangraphs (Fig 2). In [2] the waveform could be extended for the case of rotating black holes and neutron stars. In a recent publication [3], the scattering wavelets and changes in momentum and rotation due to the next-nearest-order scattering process of the gravitational constant were determined for the first time. Elaborate techniques for the calculation of Feynman integrals were used. Interestingly, the rotational degrees of freedom of black holes can be described in this new formulation by a supersymmetric world line theory [4], which is otherwise used in extensions of the standard model of particle physics.
This research takes place in the context of the DFG Research Training Group 2575 "Rethinking Quantum Field Theory", which conducts research on innovations in quantum field theory in collaboration with the MPI for Gravitational Physics and DESY.
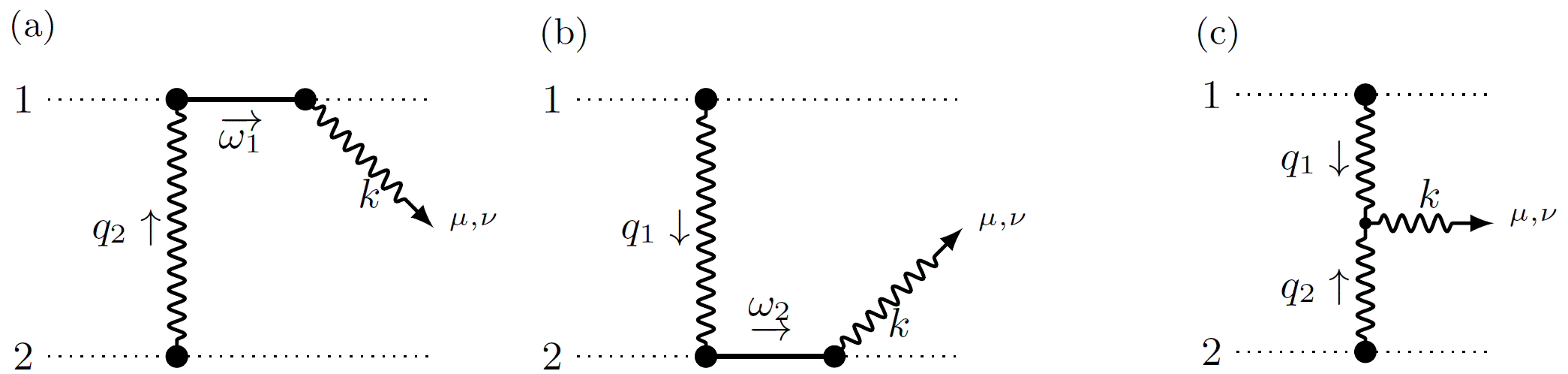
Publications:
[1] | Classical Gravitational Bremsstrahlung from a Worldline Quantum Field Theory G. U. Jakobsen, G. Mogull, J. Plefka, and J. Steinhoff Phys. Rev. Lett. 126 (2021) 201103 arxiv: 2101.12688 OPEN ![]() |
[3] | Conservative and radiative dynamics of spinning bodies at third post-Minkowskian order using worldline quantum field theory G. U. Jakobsen and G. Mogull erscheint in PRL arxiv: 2201.07778 OPEN ![]() |
|
[2] | Gravitational Bremsstrahlung and Hidden Supersymmetry of Spinning Bodies G. U. Jakobsen, G. Mogull, J. Plefka, and J. Steinhoff Phys. Rev. Lett. 128 (2022) 011101 arxiv: 2106.10256 OPEN ![]() |
[4] | SUSY in the sky with gravitons G. U. Jakobsen, G. Mogull, J. Plefka, and J. Steinhoff JHEP 2201 (2022) 027 arxiv: 2109.04465 OPEN ![]() |
More information:
Videos of Scattering Processes on Youtube (from BSc Work of O. Babayemi)
Prof. Dr. Jan Plefka
Speaker Graduiertenkolleg 2575 „Rethinking Quantum Field Theory“
Institut für Physik & IRIS Adlershof, Research Group Quantenfeld- und Stringtheorie
Email: jan.plefka-at-hu-berlin.de
Tel: +49 (0)30 2093 66409
Sekr.: +49 (0)30 2093 66413
http://qft.physik.hu-berlin.de
https://www2.hu-berlin.de/rtg2575/
Chasing an Unpredictable Quarry
Researchers explore the effects of random motion in a pursuit problem in which hounds chase a hare.
In a classic pursuit-theory problem, a pack of hounds chases a hare. A common assumption for the model is that the hare runs in a straight line or in some other prescribed way. Davide Bernardi from the Italian Institute of Technology, Ferrara, and Benjamin Lindner from Humboldt University of Berlin have added a new twist to this game: a randomly moving hare. They explore how randomness in the hare’s path and the energy constraints on the hounds affect the time it takes for the hounds to catch the hare. The results could provide guidance in developing nanorobot swimmers that can steer themselves toward a chosen goal. Pursuit theory is used in applications ranging from the designing of missile defense systems to the programming of search algorithms. Researchers have also employed the pursuit-theory framework to “microscopic chases,” in which a cell moves toward higher concentrations of some resource. Previous pursuit models included some noise in the motion, but a fully random target had not been considered until now. Bernardi and Lindner assume the hare is a Brownian particle that randomly walks over a two-dimensional surface. The hounds, by contrast, are deterministic: they always move in the direction of the hare (Fig.1). Simulations are run until one of the hounds catches up with the hare. Increasing the hare’s randomness (or diffusion rate) can delay capture, but only if the randomness is above a critical value determined by the number of hounds. In their analytical modeling, the researchers also included the energy expended by the hounds and found that—for certain consumption rates—a large number of slow-moving hounds can be more efficient than a small pack of fast-moving ones.
Figure 1: A randomly moving hare is chased by three hounds that always move in the direction of the hare.
By Michael Schirber, Corresponding Editor for Physics based in Lyon, France. Taken from https://physics.aps.org/articles/v15/s10
Publication
Run with the Brownian Hare, Hunt with the Deterministic Hounds
Davide Bernardi and Benjamin Lindner
Published on 26. January, 2022 in Physical Review Letters
Contact
Research Group Theory of Complex Systems and Neurophysics
A New Light on Quantum Science
A research team of the Institute of Physics of the Humboldt University of Berlin together with cooperation partners has, for the first time, directly measured the particle exchange phase of photons. This experiment delivers tangible proof of an astonishing quantum phenomenon which can only be observed in identical quantum objects. This represents an important step forward in quantum science.
By Lars Klaaßen
Photons are the quantum particles responsible for electromagnetic radiation; the very particles weaving the fabric of light. Any two given photons can only be distinguished if they have different wavelengths, oscillate in different directions or are located at different positions in space and time. “If two photons that are indistinguishable in wavelength and oscillation direction meet and separate again, they effectively have lost their identities” explains Kurt Busch. “Imagine we send twins through two doors into the same room. When they step out of the room, we cannot discern whether they have used the same door from which they entered or not”, completes Oliver Benson. The theory of quantum mechanics further teaches us that – according to the symmetrization postulate – two categories of elementary particles must exist: Bosons and Fermions. Members of these two families of particles can be told apart by their behavior under particle-exchange. In the twin metaphor, this corresponds to a situation in which both individuals enter and exit the two-door room by different doors. In the case of Bosons, nothing notable happens upon exchange. Fermions, on the other hand, acquire a so-called exchange phase as the wave function describing them evolves to take on an additional phase shift. “Within the twin analogy, this can be imagined as follows: If we send the twins in lockstep into the room and if they exit through different doors, they are still in lockstep. As Bosons, they step out with the same leg with which they stepped in. However, as Fermions they need to take one additional step so that they step out with the opposite leg”, says Benson. “The bosonic nature of Photons could, thus far, only be demonstrated through indirect measurements and mathematical considerations”, says Kurt Busch. “In our recent experiment we have measured the particle exchange phase for the first time in a direct manner and have thus provided direct evidence for their bosonic character.” In order to measure the exchange symmetry of a state that involves two identical particles, the team has set up an optical apparatus of the size of a small table. The setup includes a custom-made interferometer and, at the heart of it, two beam splitters. Two photons are sent through the interferometer. Impinging on the first beam splitter, they are guided along two distinct paths; in one of them, the two photons remain unaltered while in the other path they are exchanged. At the exit of the interferometer, the two photons are superimposed through the second beam splitter. “As the case may be, whether the photons are bosonic or fermionic, both photons emerge in lockstep and amplify each other or they are out of lockstep and erase themselves”, explain the physicists. Future improvements of the interferometer will provide a new tool for precision measurements with quantum light. At the same time, the experiment establishes a new method for the generation and certification of quantum states of light. This is of particular importance in the area of quantum information processing, on the basis of which novel and highly efficient computers are currently under development.
The research team of the Humboldt-Universität zu Berlin has conducted the experiment together with the Max Born Institut and the DLR-Institut für Optische Sensorsysteme.
Publication
Direct observation of the particle exchange phase of photons
K. Tschernig, C. Müller, M. Smoor, T. Kroh, J. Wolters, O. Benson, K. Busch, A. Perez-Leija
Published on 03. June, 2021 in Nature Photonics
Contact
Research Group Theoretische Optik/Photonik
Topological protection versus degree of entanglement of two-photon light in photonic topological insulators
In a joint effort, researchers from the Humboldt-Universität zu Berlin, the Max Born Institute (Berlin) and the University of Central Florida (USA), have revealed the necessary conditions for the robust transport of entangled states of two-photon light in photonic topological insulators, paving the way the towards noise-resistant transport of quantum information. The results have appeared in Nature Communications.
Originally discovered in condensed matter systems, topological insulators are two-dimensional materials that support scattering-free (uni-directional) transport along their edges, even in the presence of defects and disorder. In essence, topological insulators are finite lattice systems where, given a suitable termination of the underlying infinite lattice, edge states are formed that lie in a well-defined energy gap associated with the bulk states, i.e. these edge states are energetically separated from the bulk states, see Fig 1.
Figure 1: Topological insulators are finite-sized lattice systems (a) that exhibit eigenspectra where (b) the eigenenergies of bulk states (c) exhibit a band gap that (d) contains the eigenenergies of so-called edge states.
Importantly, single-particle edge states in such systems are topologically protected from scattering: they cannot scatter into the bulk due to their energy lying in the gap, and they cannot scatter backwards because backward propagating edge states are either absent or not coupled to the forward propagating edge states.
The feasibility of engineering complex Hamiltonians using integrated photonic lattices, combined with the availability of entangled photons, raises the intriguing possibility of employing topologically protected entangled states in optical quantum computing and information processing (Science 362, 568, (2018), Optica 6, 955 (2019)).
Achieving this goal, however, is highly nontrivial as topological protection does not straightforwardly extend to multi-particle (back-)scattering. At first, this fact appears to be counterintuitive because, individually, each particle is protected by topology whilst, jointly, entangled (correlated) particles become highly susceptible to perturbations of the ideal lattice. The underlying physical principle behind this apparent ‘discrepancy’ is that, quantum-mechanically, identical particles are described by states that satisfy an exchange symmetry principle.
In their work, the researchers make several fundamental advances towards understanding and controlling topological protection in the context of multi-particle states:
- First, they identify physical mechanisms which induce a vulnerability of entangled states in topological photonic lattices and present clear guidelines for maximizing entanglement without sacrificing topological protection.
- Second, they stablish and demonstrate a threshold-like behavior of entanglement vulnerability and identify conditions for robust protection of highly entangled two-photon states.
To be precise, they explore the impact of disorder onto a range of two-photon states that extend from the fully correlated to the fully anti-correlated limits, thereby also covering a completely separable state. For their analysis, they consider two topological lattices, one periodic and one aperiodic. In the periodic case they consider the Haldane model, and for the aperiodic case a square lattice, whose single-particle dynamics corresponds to the quantum Hall effect, is studied.
The results offer a clear roadmap for generating robust wave packets tailored to the particular disorder at hand. Specifically, they establish limits on the stability of entangled states up to relatively high degrees of entanglement that offer practical guidelines for generating useful entangled states in topological photonic systems. Further, these findings demonstrate that in order to maximize entanglement without sacrificing topological protection, the joint spectral correlation map of two-photon states must fit inside a well-defined topological window of protection, Fig. (2).
Figure 2: In order to identify the topological window of protection, the researchers considered a spectrally broad product state as initial state and propagate it through an ensemble of 1000 random Haldane lattices. (a) Depicts the spectral correlation map for the initial state and in (b) the ensemble-average of the spectral correlation maps inside the edge-edge subspace after the propagation through the ensemble of disordered lattices is shown. It is found that the only two-photon amplitudes that survive the scattering induced by the disorder lie in the region indicated by the black square which is the protection window. Finally, (c) and (d) display, respectively, the edge-mode content E and the product of the edge-mode content with the Schmidt-number E · SN as a function of the variances of the initial states.
Publication
Konrad Tschernig, Álvaro Jimenez-Galán, Demetrios N. Christodoulides, Misha Ivanov, Kurt Busch, Miguel A. Bandres, Armando Perez-Leija
Publication 30 March 2021, Nature Communications 12, Article number: 1974 (2021)
Contact
Theoretical Optics & Photonics Group
Stability of non-supersymmetric solutions of string theory
One of string theory's most prominent predictions is that our universe has extra spatial dimensions that we do not observe directly. Instead, these extra dimensions are curled up into a small compact space. In order to yield any meaningful physics, these "compactifications" must be stable, much like a ball in a valley of a hill is stable since if it is pushed slightly to the side, it will roll-back to its initial position. By contrast, if the ball is at the top of a hill, a small push to the side will cause the ball to roll down the hill and far away from its original unstable position. Similarly, in an unstable compactification, a small deformation of the shape of the extra dimensions of string theory will cause the extra dimensions to rapidly expand to large size or even to collapse and form a rip in space. Typically, string compactifications are unstable, unless they possess supersymmetry, an elegant symmetry arising in string theory but which is not observed in the real world.
Adolfo Guarino (University of Oviedo), Emanuel Malek (Humboldt-Universität zu Berlin) and Henning Samtleben (École Normale Supérieure de Lyon) proved the stability of 7 non-supersymmetric solutions of string theory, consisting of a 4-dimensional negatively curved spacetime, called anti-de Sitter space, and 6 curled up dimensions making up a spherical shape. These form the first examples of stable string theory solutions containing anti-de Sitter space. While these solutions cannot be used to directly model our universe, they could provide the first models for 3-dimensional non-supersymmetric condensed matter systems via the "holographic principle". Moreover, this class of solutions are a stepping stone towards better understanding non-supersymmetric solutions of string theory in general.
Publication
Stable Nonsupersymmetric Anti–de Sitter Vacua of Massive IIA Supergravity
Adolfo Guarino, Emanuel Malek, Henning Samtleben
Publication 10 February 2021, Phys. Rev. Lett. 126 (2021) 061601
Contact
Emmy Noether Research Group "String Theory Flux Vacua"
Sharing is Caring! Discovering the materials of the future with FAIR data management and AI
Panel discussion with Prof Claudia Draxl (HU) and Prof Matthias Scheffler (HU and Fritz Haber Institute of the Max Planck Society), moderated by Prof Peter Frensch (HU, Vice President for Research) at the Berlin Science Week 2020
Many products owe their function to novel materials, but how can we find materials that perform particularly well and enable new technologies? By making smarter use of our knowledge!
Huge amounts of scientific data are generated worldwide, but much of it is considered waste because this data does not serve the specific research project. However, it could contain valuable information for other research approaches. This calls for a rethink: Recycle the Waste!
At this year's Berlin Science Week, Claudia Draxl and Matthias Scheffler demonstrated that this approach can only be realized with Artificial Intelligence and a FAIR data infrastructure. (FAIR stands for Findable, Accessible, Interoperable, and Re-purposable.) Such an infrastructure enables the productive handling of scientific data and is thus essential for the development of future technologies.
Draxl and Scheffler have already established such an infrastructure for computational materials science: The NOMAD Lab is the world's largest database of material properties, which now contains 100 million calculations. Draxl and Scheffler are even going one step further. As part of the NOMAD Center of Excellence, they are developing computational materials science into new applications, preparing it for the coming generation of high-performance computers (exascale computers).
More Information
Video of the panel discussion in German
Contact
The Novel Materials Discovery (NOMAD) Laboratory
The NOMAD Center of Excellence
Density fluctuations in amorphous silicon discovered
For the first time, it has been possible to identify atomic substructures in amorphous silicon with a resolution of 0.8 nanometres using X-ray and neutron scattering at BESSY II and BER II. Such a-Si:H thin films have been used for decades in solar cells, TFT displays, and detectors. The results show that three different phases form within the amorphous matrix, which dramatically influences the quality and lifetime of the semiconductor layer. The structural elucidation was achieved in a collaboration between the Department of Physics of the HU, the Helmholtz-Zentrum Berlin and the Technical Universities of Eindhoven and Delft.
Silicon does not have to be crystalline, but can also be produced as an amorphous thin film. If additional hydrogen is incorporated during the production of these thin layers, so-called a-Si:H layers are formed. Such a-Si:H thin films have been used for decades for various applications, for example as contact layers in highly efficient tandem solar cells made of perovskite and silicon. In this study, it was shown that the a-Si:H network is by no means a homogeneously amorphous material. The amorphous matrix is interspersed with nanometre-sized areas of varying local density, from cavities to areas of extremely high order. It was possible to experimentally observe and quantitatively measure these inhomogeneities in differently produced a-Si:H thin films. To do this, the results of complementary analytical methods were combined to form an overall picture.
At the nanometer scale, voids corresponding to slightly more than 10 missing atoms were discovered. These voids arrange themselves with a distance of about 1.6 nanometres. In addition, nanometre-sized regions were discovered in which silicon atoms are better ordered compared to the surrounding material. These densely ordered domains (DOD) contain hardly any hydrogen. The DODs form aggregates of up to 15 nanometres in diameter. The DOD regions are able to reduce mechanical stress in the material and thus contribute to the stability of the a-Si:H thin film. The voids on the other hand, can promote electronic degradation of the semiconductor layers
Targeted optimization of manufacturing processes with regard to the substructures now discovered could enable new applications such as optical waveguides for programmable photonic systems or a future silicon battery technology.
Structural model of highly porous a-Si:H, which was deposited very quickly, calculated based on measurement data. Densely ordered domains (DOD) are drawn in blue and cavities in red. The grey layer represents the disordered a-Si:H matrix. The round sections show the nanostructures enlarged to atomic resolution (below, Si atoms: grey, Si atoms on the surfaces of the voids: red; H: white) Figure: Eike Gericke/HZB
Publication
Quantification of nanoscale density fluctuations in hydrogenated amorphous silicon
Eike Gericke, Jimmy Melskens, Robert Wendt, Markus Wollgarten, Armin Hoell, Klaus Lips
Publication 29 October 2020 Phys. Rev. Lett. 125 (2020) 18, 185501
Print Version of the Cover (pdf)
Contact
Prof. Simone Raoux, Institute for Nanospectroscopy, HZB
Quantum microscopy reveals invisible bio-features
Researchers from Humboldt-Universität zu Berlin and the Experimental and Clinical Research Center in Berlin show how quantum light can help the field of bioimaging. In their new experiment, featured on the cover of "Science Advances", the team uses entangled photons to image a bio-sample probed by "invisible" light without ever looking at that light. This avoids the usually severe problems that stem from poor performance and high price of broadband mid-IR light sources and cameras. Instead, the researchers only use a normal laser and commercial CMOS camera. This makes their mid-IR microscopy technique not only robust, fast and low noise, but also cost-effective - making it highly promising for real-world applications. They show this by taking microscopic images of a tissue sample from a mouse heart.
Figure: Quantum microscopy of a mouse heart. Entangled photons allow for the making of a high-resolution mid-IR image, using a visible light (CMOS) camera and ultralow illumination intensities. In the picture, absorption (left) and phase information (right) from a region in a mouse heart. The yellow scale bar corresponds to 0.1 mm which is about the width of a human hair.
More Information
News of the Humboldt-Universität zu Berlin
Publication
Microscopy with undetected photons in the mid-infrared
Inna Kviatkovsky, Helen M. Chrzanowski, Ellen G. Avery, Hendrik Bartolomaeus, Sven Ramelow
Publication 14 October 2020, Science Advances 6, Issue 42
Contact
Nonlinear Quantum Optics Group
Hidden Symmetries in Massive Quantum Field Theory
Theoretical models with a large amount of symmetry are ubiquitous in physics and often key to developing efficient methods for complex problems. If the number of symmetries surpasses a critical threshold, a system is called integrable with a prime example being the Kepler problem of planetary motion. While integrability typically comes with a rich spectrum of mathematical methods, it is often hard to identify the underlying symmetries. For the first time quantum integrability was now discovered in the context of massive quantum field theories in four spacetime dimensions. Florian Loebbert and Julian Miczajka (both Humboldt University) together with Dennis Müller (NBI Copenhagen) and Hagen Münkler (ETH Zürich) have shown that large classes of mostly unsolved massive Feynman integrals feature an infinite dimensional Yangian symmetry - a hallmark of integrability. This mathematical structure is highly constraining and it allows to completely fix these building blocks of quantum field theory as has been demonstrated for first examples. The observed Yangian symmetry goes hand in hand with an extension of the important structure of conformal symmetry to situations including massive particles. Remarkably, this discovery suggests that similar symmetry features may also be hidden in massive versions of the celebrated holographic duality between gauge theories and gravity. These findings were recently published in Physical Review Letters 125 (2020) 9, 091602.
Publication
Massive Conformal Symmetry and Integrability for Feynman Integrals
Florian Loebbert, Julian Miczajka, Dennis Müller, and Hagen Münkler
Publication 25 August 2020, Phys. Rev. Lett. 125 (2020) 9, 091602
Contact
Quantum Field and String Theory Group
Jetting into the dark side: a precision search for dark matter
The nature of dark matter remains one of the great unsolved puzzles of fundamental physics. Unexplained by the Standard Model, dark matter has led scientists to probe new physics models to understand its existence. Many such theoretical scenarios postulate that dark matter particles could be produced in the intense high-energy proton–proton collisions of the LHC. While the dark matter would escape the ATLAS detector unseen, it could occasionally be accompanied by a visible jet of particles radiated from the interaction point, thus providing a detectable signal.
The ATLAS Collaboration set out to find just that. Today, at the International Conference in High-Energy Physics (ICHEP 2020), ATLAS presented a new search for novel phenomena in collision events with jets and high missing transverse momentum (MET). The search was designed to uncover events that could indicate the existence of physics processes that lie outside the Standard Model and, in doing so, open a window to the cosmos.
A monojet event recorded by the ATLAS experiment in 2017, with a single jet of 1.9 TeV transverse momentum recoiling against corresponding missing transverse momentum (MET). The green and yellow bars show the energy deposits in the electromagnetic and hadronic calorimeters, respectively. The MET is shown as the red dashed line on the opposite side of the detector. (Image: ATLAS Collaboration/CERN)
More Information
Physics Briefing, By ATLAS Collaboration, 27th July 2020
Contact
Groups: Experimental Particle and Astroparticle Physics and High Energy Physics
Direct measurement of quantum efficiency of single-photon emitters in hexagonal boron nitride
Single-photon emitters (SPEs) in two-dimensional materials are promising candidates for the future generation of quantum photonic technologies. In this work, we experimentally determine the quantum efficiency (QE) of SPEs in few-layer hexagonal boron nitride (h-BN). We employ a metal hemisphere that is attached to the tip of an atomic force microscope to directly measure the lifetime variation of the SPEs as the tip approaches the ℎ-BN. This technique enables nondestructive, yet direct and absolute measurement of the QE of SPEs. We find that the emitters exhibit very high QEs approaching (87±7)% at wavelengths of ≈580 nm, which is among the highest QEs recorded for a solid-state SPE
Schematics of an experiment to measure the quantum efficiency of a single photon emitter in a two-dimensional material: A metal sphere very close to the emitter changes the spontaneous emission rate due to a quantum electrodynamic effect thus revealing its quantum efficiency.
Publication
Direct measurement of quantum efficiency of single-photon emitters in hexagonal boron nitride
Niko Nikolay, Noah Mendelson, Ersan Özelci, Bernd Sontheimer, Florian Böhm, Günter Kewes, Milos Toth, Igor Aharonovich, and Oliver Benson
Optica 6 (2019), 1084-1088
Print Version of the Cover (pdf)